Menu (linked Index)
Metabolic Pathways
Last Update: October 31, 2024
- Introduction
- Metabolism Defined
- Cellular Respiration
- Central Metabolism – Proteins, Fats
- Metabolic Routes
- Pentose Phosphate Pathway
- Glycogen Pathway
- Fat Metabolism Path
- Lactic Acid Glucose Pathway
- Protein Metabolism Paths
- Acetyl CoA Metabolic Routes
- Summary of Biosynthesis Routes
- Tissue Specific Metabolic Routes
- Conclusion
Introduction
The chemical reactions that run and maintain life are varied and often interrelated. Collectively we describe these as Metabolism.
The core mechanism of Metabolism is the Cellular Respiration reaction pathway.
It is sometimes described as Central Metabolism because practically all metabolic paths for different nutrients pass through it or are connected to it in some way.
That is, Carbohydrates, Proteins, and Fats can all undergo Cellular Respiration to produce energy, but they can all be used to create molecules as well.
In this post we’ll review the Cellular Respiration pathway and then show how other metabolic processes are connected and related to it.
Metabolism Defined
Picture_Metabolism – The Chemical Reactions that Sustain Life
Summarizing the picture above (see Intro to Metabolism, Anabolism, Catabolism – www.khanacademy.org):
- Metabolism consists of catabolic processes that break down nutrients and anabolic processes that build them back up.
- Cellular Respiration breaks down Glucose (and other nutrients depending on the current “state” of the body) to produce energy.
- Cellular Respiration Overall reaction:
- C₆H₁₂O₆ (Glucose) + 6 O₂ (Oxygen) = 6 CO₂ (Carbon Dioxide) + 6 H₂O (Water) + ~32 ATP (containing readily usable energy)
- Oxygen is needed (although anaerobic routes also occur to a lesser degree).
- Carbon dioxide ,water, and the molecule ATP are created.
- Cellular Respiration provides the energy needed to create ATP in a reaction called Phosphorylation (the enzyme ATP Synthase enables this to happen).
- ATP (Adenosine TriPhosphate), enabled by various enzymes, can release a phosphate group, release energy, and convert to ADP (Adenosine DiPhosphate).
- This released energy is used to power cellular processes (both in anabolic and catabolic processes).
- And the cycle continues, with ATP being produced from ADP via cellular respiration etc..
Cellular Respiration
The picture below is a high level reaction pathway schematic for the Cellular Respiration of Glucose in the human body.
Remarkably it’s very similar in most other living organisms as well!
Glucose is the key micronutrient that our body relies on to survive (it is a breakdown product of carbohydrates).
For example, our brain primarily relies on Glucose for energy.
“What about the protein and fat we eat?”, you might ask.
These can also be used for energy (they are also key ingredients in many anabolic reactions) and can enter (or interact with components of) this respiration pathway in different ways.
This is why the Cellular Respiration pathway is described as Central Metabolism (practically all metabolic paths for different nutrients pass through it in some way).
But let’s start with a description of Glucose Cellular Respiration.
Picture_Central Metabolism (Cellular Respiration)
- You consume, digest, and “process” food with the assistance of oxygen.
- When you breath out, you are rejecting CO2 from your body.
- intermediate molecules (NADH and FADH2) and
- also carried in the ultimate desired final molecule, ATP (about 27 – 38 molecules are made for each molecule of Glucose although 32 is often stated as the average number).
Glucose Cellular Respiration Occurs in 4 stages
Refer to the drawing above as you read the following.
- Glycolysis breaks down 1 Glucose into 2 Pyruvates and generates energy carrying molecules like NADH and ATP.
- In a relatively simple Transition Step, Pyruvate converts to Acetyl CoA which enters the Krebs cycle.
- The Krebs Cycle builds up and breaks down molecules to generate more energy carrying molecules like NADH, FADH2, and ATP.
- This cycle also creates building block intermediates for other molecules.
- Oxidative Phosphorylation takes the energy from NADH and FADH2 and creates (a lot) more ATP. It involves:
- Oxidation of NADH and FADH2,
- Activation / Energization of a Chemiosmotic process by
- electrons cascading through an Electron Transport Chain (ETC) of proteins, and
- Phosphate addition to ADP to make ATP
Glycolysis occurs in the Cytoplasm of a cell while the Krebs Cycle and Oxidative Phosphorylation occur in the Mitochondria.
Picture_Cellular Respiration in the Cell
So How much ATP is Actually Produced?
The literature is not 100% consistent on the number of ATP produced per molecule of NADH and FADH2 (it’s an ongoing area of research).
The following numbers are based on Khanacademy.org , NIH and a few other sources:
- 4 ATP produced directly from Glycolysis and the Krebs Cycle
- 23 to 34 ATP From Oxidative Phosphorylation
- About 2 to 3 ATP per NADH2 (so “10 x 2 = 20” to “10 x 3 = 30” ATP produced)
- About 1.5 to 2 ATP per FADH2 (so “2 x 1.5 =3” to “2 x 2 =4” ATP produced)
- So a total of 23 to 34 ATPs are produced from Oxidative Phosphorylation
- Total: 27 to 38 ATP from one Glucose molecule.
Sometimes 30 – 32 ATP is suggested as the actual range (or just 32 sometimes!).
Don’t get too concerned about the exact number; there probably isn’t one since the efficiency of the NADH2 and FADH2 conversions to ATP can vary.
Check Out My Cellular Respiration Series for more Information!
Central Metabolism (Proteins, Fats)
Glucose is not the only chemical the body utilizes for energy.
So, how do the non-Glucose nutrients get metabolized by the body”?
Answer: Practically all metabolic paths for different nutrients “touch” or pass through the Cellular Respiration pathway.
This Central Metabolism concept is so important.
Glucose, you recall, is the main breakdown product of carbohydrate foods.
Below I present a simplified picture of Glucose Cellular Respiration which we’ll “build upon” a little later on in this post.
Picture_Cellular Respiration Simplified Flow Chart
Now, how do Fats and Proteins get processed and can they be related to the chart above somehow?
- Fat we consume is digested mainly into Glycerol and Fatty Acids
- It is a kind of lipid.
- Proteins we eat are broken down into Amino Acids
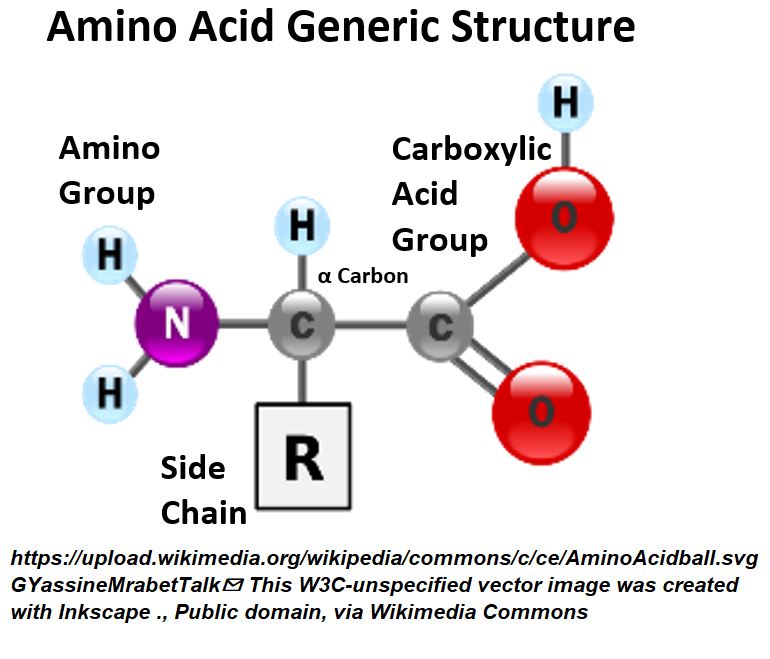
- The actual reaction pathways are complex and still being discovered and described.
- Each step involves interactions among enzymes and hormones and other “helper molecules”,
- all converging, diverging, or otherwise interacting with this Super-Metabolic-Highway of the body called Cellular Respiration.
- My goal is to give you a “sniff” of some of the amazing ways in which your body integrates all these reactions.
- Be amazed and find yourself a good biochemistry text or a good online reference if you want to learn more
Metabolic Routes
I took the basic Cellular Respiration drawing shown in the previous section and added some other metabolic processes to it.
You get the picture below.
We have the same Cellular Pathway skeleton (Glucose -> Pyruvate -> Acetyl CoA -> Krebs -> Oxidative Phosphorylation), but now I show other reactions that can occur.
- A black line means a “Fed Metabolic State” where a person’s energy demands are satisfied (i.e. you are fed or full of food).
- A red line represents a “Fasting State” where the body is in need of energy.
- In reality , most of these are probably happening all the time, but to a lesser or greater degree.
Picture_Metabolic Routes Chart
You can refer to this picture as we describe some of these metabolic process in the following sections.
Pentose Phosphate Pathway
This pathway begins near the top of the Glycolytic pathway, with the intermediate, Glucose-6-phosphate (G6P). It produces
- NADPH.
- NADPH is Nicotinamide Adenine Dinucleotide Phosphate
- It is similar structurally to NADH.
- NADPH is an electron carrier involved in many synthesis (anabolic) reactions.
- e.g. Fat Acid, Cholesterol, Steroid and other synthesis reactions
- Ribose-5-Phosphate.
- It is used to synthesize nucleotides, which, as we have seen, are the building blocks of DNA and RNA.
The Pentose Phosphate Pathway can occur in various metabolic states.
References:
Glycogen Pathway
This pathway also begins near the top of the Glycolytic pathway, with the intermediate, Glucose-6-phosphate.
Glycogen is a temporary storage form of Glucose.
In order to reduce blood sugar, the hormone Insulin will cause Glucose and Glucose intermediates to polymerize into Glycogen.
In order to raise blood sugar, the hormone Glucagon will cause the release of Glucose from Glycogen.
Glycogen is essentially a collection of Glucose like molecules arranged in a polymer structure.
- It is stored mostly in the liver and skeletal muscle.
- The Glycogen to Glucose interconversion to maintain blood Glucose levels happens mainly in the liver.
The hormones Insulin and Glucagon work together to maintain a target range of blood Glucose.
- Your Brain is a big consumer of your daily Glucose intake (60% to 70%)
- 70 to 120 mg/dL (milligrams per deciliter) Glucose in blood is the typical blood regulation range.
- >126 mg/dL makes you a Diabetic (Extreme Hyperglycemia)
- < 40 mg/dL makes you Hypoglycemic (Subject to fatigue or even death)
- Pancreatic Insulin increases the uptake of Glucose by our cells to promote Glycolysis.
- Glycogenesis is the production of Glycogen from Glucose (and Glucose intermediates)
- Glucose (intermediates) –> Glycogen
- This is a a (temporary) Glucose storage mechanism.
- It is stimulated by the hormone Insulin.
- Insulin is released by the Pancreas when blood sugar is high.
- Insulin inhibits the release of the hormone Glucagon (read below).
Glycogenolysis is the breakdown of Glycogen to Glucose and Glucose intermediates
- Glycogen (Glucose Polymer) –> Glucose (intermediates)
- It is a Glucose release mechanism.
- It is stimulated by the hormone Glucagon.
- Glucagon is released by the Pancreas when blood sugar is low.
- It’s also stimulated by the hormone Epinephrine (Adrenaline).
- Epinephrine is released by the Adrenal Glands in response to stress or physical activity.
Fat Metabolism Path
When we say we eat “Fat”, we mean “Lipids“, which include
- Fats (Triglycerides), Phospholipids , Cholesterol, and certain Vitamins.
We are going to focus on Triglycerides (AKA Triacylglycerides or TAG)
- A Similar digestion process occurs for phospholipids while
- cholesterol and fat soluble vitamins are not enzymatically broken down.
An example Fat (TAG) molecule is shown in the picture below.
Picture_Triglyceride
TAGs have a hydrophilic (water loving) Glycerol Backbone and 3 hydrophobic (water repelling) Fatty Acid Chains.
- In the picture above, the TAG consists of 3 Fatty Acid Chains
- Palmitic Acid
- Oleic Acid
- Alpha-Linolenic Acid
Metabolic Path and Disposition of Dietary Fat
- See this Really nice material on fat transport in body.
- Fats in the Stomach tend to form globules and don’t dissolve adequately for efficient digestion
- The majority of digestive enzymes and Bile Acids are secreted into the small intestines
- Bile acids emulsify fats into smaller droplets, increasing their surface area.
- Bile acids are produced in the Liver and stored in the Gallbladder.
- Reduced Fat particle sizes (greater surface areas) means more efficient enzymatic action.
- Enzymes (mainly Lipase produced by the Pancreas) break down Fats into Fatty acids and Glycerol.
- Glycerol and Palmitic Acid (a Fatty Acid) structures are shown below.
Picture_Glycerol and Palmitic Acid (a Fatty Acid)
Fatty Acids and Glycerol are absorbed into the intestinal cells.
- The smaller sizes of these molecules (relative to the TAGs) facilitate the absorption.
- They recombine to form Triglycerides.
- It seems inefficient to break down TAGs and then recombine them but,
- the subsequent recombination allows for efficient transport through the body.
- Triglycerides (TAGs), along with Cholesterol and Phospholipids, are packaged into Chylomicrons.
- These Lipoproteins enter the Lymphatic System and eventually the bloodstream.
- Chylomicrons transport fats to various tissues (adipose tissue for storage and muscle cells for energy).
Check out the picture of a Chylomicron below. These are complex spherical Lipoproteins consisting of
- A (mostly) Phospholipid surface layer,
- a cargo of mostly TAGs, and
- embedded Apolipoproteins (or Apo).
There are other types of Lipoproteins (LDL, HDL, VLDL) that also carry Lipids including Cholesterol. They are mainly differentiated by the types of embedded Apolipoproteins.
- The terms Good and Bad Cholesterol refer primarily to the LDLs and HDLs.
- This is a bit misleading since Cholesterol is carried in both types.
- LDLs are considered bad because they can stick to blood vessel walls and cause Atherosclerosis.
Picture_Chylomicron: A type of Lipoprotein
In the body, Triglycerides are once again broken down into Fatty Acids and Glycerol.
- Fatty Acid Interconversion:
- In a “Fasting Metabolic State”, Fatty Acids are converted to Acetyl CoA (by Beta-Oxidation) in the Mitochondria.
- Acetyl-CoA can then enter the Krebs Cycle and the Electron Transport Chain (to produce ATP = Energy),
- Acetyl-CoA can also react to create Ketone Bodies (Ketogenesis: another form of energy used by Brain mainly)
- Ketone bodies (Acetone, Acetoacetic acid, and Beta-hydroxy butyric acid) are transported in the blood mainly to the brain
- where they convert back to Acetyl CoA and generate ATP.
- In a “Fed Metabolic State” Acetyl CoA can convert back to Fatty Acids (Fatty Acid Synthesis).
- Glycerol Interconversion:
- Glycerol can be converted to Glucose (Gluconeogenesis) in a Fasting Metabolic State.
- In Gluconeogenesis , Glycerol enzymatically converts to DHAP which is a Glycolysis reaction intermediate.
- From this entry point it follows an enzymatically driven path to Glucose (Blue Line in picture below)
Picture_Glycerol to Glucose Reaction Pathway
- Glycerol can convert to Triglycerides or TAGs (Lipogenesis) in a Fed Metabolic State.
- Hormone Regulation:
- Insulin and Glucagon are the big players here.
- Insulin promotes conversion of Glucose to Glycerol.
- Insulin promotes Fatty Acid Synthesis and ultimately lipid production (Lipogenesis).
- Glucagon promotes Glucose production via Gluconeogenesis (via Glycerol)
- Glucagon promotes Fatty Acid Oxidation and production of Ketone Bodies (Ketogenesis).
- Epinephrine (Adrenaline) Promotes fatty acid oxidation.
- There are others.
In summary, Lipids are
- primarily digested in the small intestines,
- absorbed into the bloodstream by way of the Lymphatic system,
- transported to the body’s tissues, and
- used for immediate energy use or stored for future use (as fat in adipose tissue mainly).
Lactic Acid to Glucose Pathway
Lactic Acid, produced anaerobically from Pyruvate, can enzymatically convert back to Pyruvate, and ultimately back to Glucose via Gluconeogenesis
Picture_Lactic Acid to Glucose Pathway
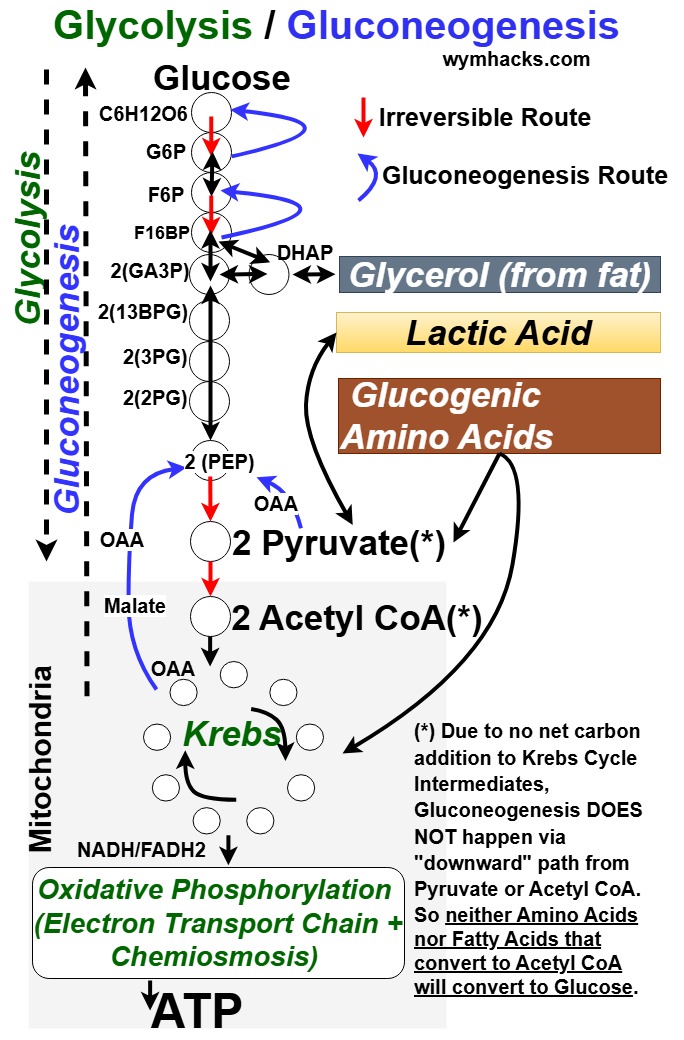
Lactic Acid Formation
During intense exercise (limited oxygen supply), muscle cells switch to anaerobic metabolism.
- Glucose is broken down into Pyruvate through Glycolysis.
- Pyruvate is converted to Lactate by the enzyme Lactate Dehydrogenase.
- This helps regenerate NAD+ for continued Glycolysis.
- Lactic acid can accumulate in muscle tissue (causing muscle fatigue).
Lactic Acid to Glucose (Gluconeogenesis)
In Lactate Gluconeogenesis, the following steps occur:
- Lactate is enzymatically oxidized back to Pyruvate , regenerating NAD+.
- Pyruvate is enzymatically carboxylated to Oxaloacetate (OAA).
- Oxaloacetate (OAA) is enzymatically decarboxylated and phosphorylated to Phosphoenolpyruvate (PEP).
From PEP, the pathway follows the reverse steps of glycolysis, following the enzymatically driven “blue path” shown in the drawing.
This primarily occurs in the liver where Glucose can be released into the bloodstream to provide energy as needed.
Protein Metabolism Paths
- Protein Digestion
- In the Stomach, Proteins are broken down into smaller polypeptides by Hydrochloric Acid and the enzyme Pepsin.
- In the Small Intestine Pancreatic enzymes break down polypeptides into smaller peptides and Amino Acids.
- Small Intestine Lining enzymes complete the breakdown of peptides into individual Amino Acids.
- Amino Acids are absorbed through the intestinal walls into the bloodstream.
- Protein Synthesis
- Amino Acids build and repair essential tissues, enzymes, hormones, and proteins.
- Some Amino Acids are precursors for the synthesis of
- neurotransmitters,
- heme groups in hemoglobin ,
- and nucleotides (used to synthesis the nitrogenous bases).
- Energy Production: Amino Acids can be
- converted into Glucose (Gluconeogenesis via Glucogenic Amino Acids) or
- Ketone Bodies (via Ketogenic Amino Acids) to provide energy.
- This process involves Deamination, where the amino group is converted to Urea (in Liver) and excreted through the Kidneys.
Appreciate the various amino acid routes in the picture below!!
Picture_Amino Acid Metabolic Routes
Picture_Glucogenic Amino Acids to Glucose Pathways
- Amino Acid Routes:
- Amino Acids can convert to Keto Acids or directly to Acetyl CoA.
- Keto Acid examples are: Pyruvate, Alpha Ketoglutarate, and Oxaloacetate.
- Keto Acids that are Krebs Cycle intermediates can enter the Cellular Respiration path and produce energy.
- Ketogenic Amino Acids ultimately convert to Ketone Bodies (via Acetyl CoA mainly; Called Ketogenesis).
- Ketone bodies (Acetone, Acetoacetic Acid, and Beta-Hydroxy Butyric Acid) are transported in the blood mainly to the brain
- where they convert back to Acetyl CoA and generate ATP.
- Glucogenic Amino Acids can convert back to Glucose via Gluconeogenesis by way of
- Pyruvate or Krebs Cycle Intermediates.
- So, in Keto Acid form, Amino Acids can participate directly (starting in the Krebs Cycle) in Cellular Respiration or be converted to Glucose via Gluconeogenesis.
- Hormonal Regulation of Amino Acid Metabolism
- Insulin promotes protein synthesis and uptake by cells,
- Glucagon (and Cortisol) stimulate protein breakdown and amino acid conversion to Glucose (via Gluconeogenesis)
- Growth , Thyroid Hormones, and Sex Hormones promote protein synthesis and growth.
Reference:
Acetyl CoA Metabolic Routes
Picture_Acetyl CoA Metabolic Routes from Central Metabolism Hub
Note: The following text (in quotations below) was generated with Google Gemini:
Acetyl CoA and Fatty Acids
“Fatty acids, the building blocks of lipids, undergo a dynamic cycle of breakdown and synthesis.
In fatty acid oxidation, long-chain fatty acids are broken down into two-carbon units of acetyl-CoA through a process called beta-oxidation.
- This process occurs in the mitochondria and generates energy in the form of ATP.
Conversely, fatty acid synthesis involves the conversion of acetyl-CoA into fatty acids.
- This process takes place in the cytoplasm and requires energy in the form of NADPH.
The newly synthesized fatty acids can be used for various purposes:
- Triacylglycerol (TAG) synthesis: Fatty acids combine with glycerol to form triglycerides, the primary storage form of energy in the body.
- Phospholipid synthesis: Fatty acids are essential components of phospholipids, which are major constituents of cell membranes.
- Myelin sheath formation: Fatty acids, particularly long-chain polyunsaturated fatty acids, are crucial for the formation and maintenance of the myelin sheath, a protective layer around nerve cells.”
Acetyl CoA and Cholesterol
“Acetyl-CoA, …, is the building block for cholesterol.
Through a complex series of reactions, it is converted into cholesterol, a vital molecule for various cellular functions.
Cholesterol serves as a precursor for several essential substances:
- Vitamin D: Upon exposure to sunlight, cholesterol in the skin is converted into vitamin D, which plays a crucial role in calcium absorption and bone health.
- Steroid Hormones: Cholesterol is the starting material for the synthesis of steroid hormones, including estrogen, testosterone, cortisol, and aldosterone.
- These hormones regulate various physiological processes, such as reproduction, stress response, and electrolyte balance.
- Bile Acids: Cholesterol is converted into bile acids in the liver, which aid in the digestion and absorption of fats in the small intestine.”
Acetyl CoA and Ketone Bodies
“Acetyl-CoA, …, can be converted into ketone bodies during periods of low Glucose availability, such as fasting or prolonged exercise.
This process, known as ketogenesis, primarily occurs in the liver.
Ketone bodies, including acetoacetate, beta-hydroxybutyrate, and acetone, are water-soluble and can be transported through the bloodstream to tissues like the brain and heart.
- These tissues can utilize ketone bodies as an alternative energy source, especially when Glucose levels are low.
- Ketone bodies are particularly important for the brain, which has limited capacity to use fatty acids directly as fuel.”
Note: The following text (in quotations above) was generated with Google Gemini.
Summary of Biosynthesis from Central Metabolism
I hope it’s clear how Glycolysis and the Krebs Cycle and Oxidative Phosphorylation are at the center of metabolism in the body (We call this Central Metabolism).
This makes sense since ATP is produced and helps energize the body’s various biological reactions.
The chart below is an incomplete summary just showing examples of biosynthesis in the body that keys off of Central Metabolism.
Biosynthesis Routes from Central Metabolism
As Godly as this process is, it doesn’t cover everything we need to survive.
- We can’t make Vitamins and we need to get these from our diet.
- We can’t make Minerals as well (sodium, magnesium, potassium, chromium etc.) and must get them from our diet
- Read my post on Vitamins and Minerals
References:
Tissue Specific Metabolism Paths
Picture_Tissue Specific Metabolism (Version 1)
Picture Tissue Specific Metabolism (Version 2)
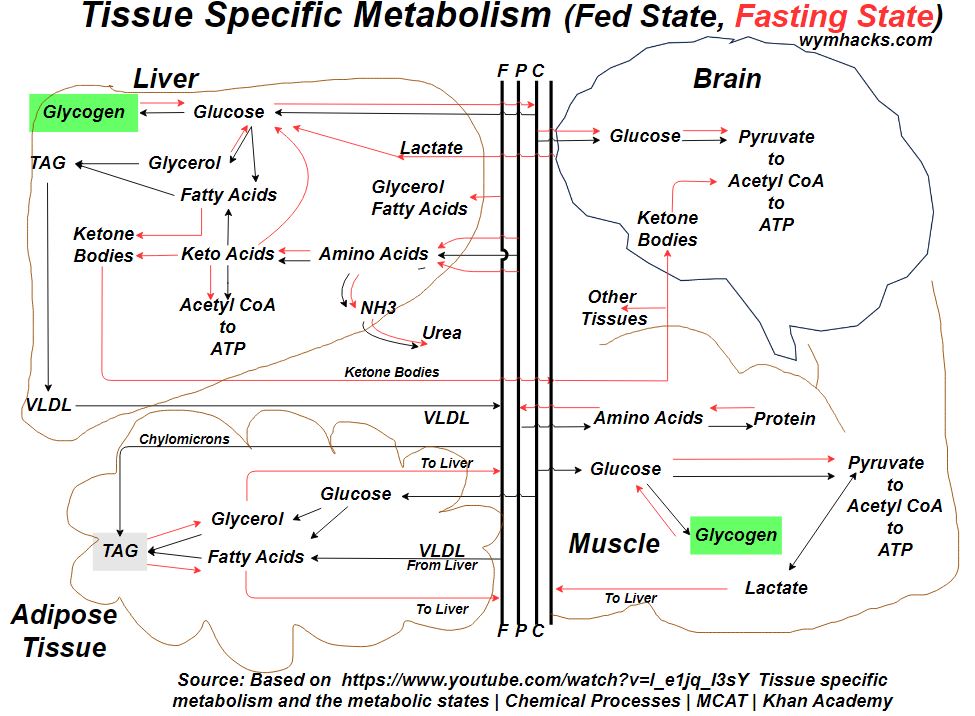
Conclusion
The Cellular Respiration Pathway serves as a central hub for several critical biochemical reactions.
It allows you to extract energy not only from carbohydrates (e.g. Glucose) but also from Proteins (Amino Acids) and Fats (Glycerol and Fatty acids).
Cellular Respiration Pathway intermediates also play critical roles in anabolic processes (i.e. building molecules needed for life like tissues, organs, enzymes, hormones etc.)
On the surface it seems relatively simple…You ingest nutrients and oxygen and you reject carbon dioxide, water, and waste.
But all the time, an incredibly complicated blend of biochemical reactions are going on in your body.
The reactions are tightly regulated and controlled (via enzymes and hormones) to ensure your body stays alive and nourished.
Disclaimer: The content of this article is intended for general informational and recreational purposes only and is not a substitute for professional “advice”. We are not responsible for your decisions and actions. Refer to our Disclaimer Page.